Share this
Polymer Pyramid: Choose the Best 3D Printing Material
by Gabriela Greste on Oct 4, 2024 5:46:20 PM
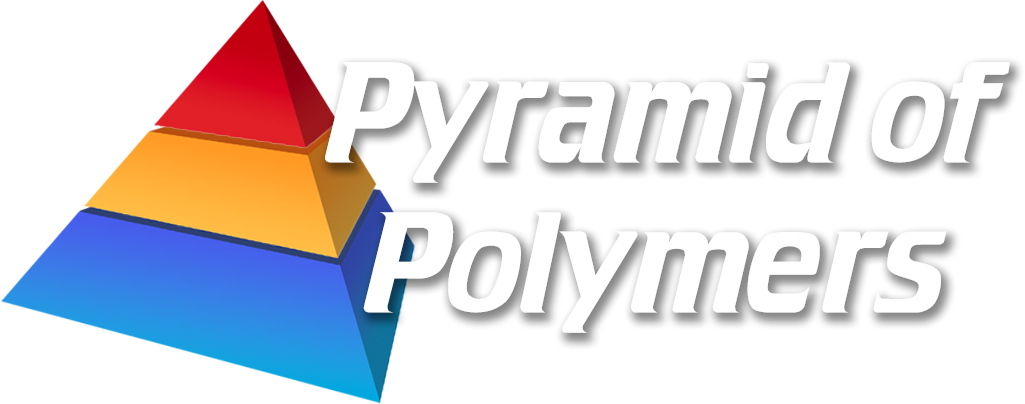
Introduction to Polymers and 3D Printing
Before 3D Printing, choosing the right material for your project can make or break it. There are hundreds of Polymers available on the market with thousands of combinations so selecting the ideal one can be challenging for anybody. Fortunately, we here at 3devo use a model called the Polymer Pyramid or Plastics Pyramid that can help you easily choose your material. This guide will help innovators and engineers decide which plastic to use, whether you are developing a prototype or recycling plastic waste.
Polymer Materials and Processability
They appear in everyday life from basic packaging products to highly specialized applications. Whether you’re 3D printing or creating new filament materials, understanding a polymer’s characteristics—like its chemical resistance, thermal resistance, and mechanical properties—will be crucial to the match the planned needs and outcome of your project. Furthermore, other key factors such as thermal coefficient, additive percentage and melt viscosity will be essential when choosing a material grade. These properties help us determine the material's suitability for specific applications but also its processability, affecting how easily it can be extruded into filament and the final print quality.
Selecting the right polymer is about finding a balance between your project’s requirements and the material’s behaviour during the extrusion process.
Molecular Structure and Material Behavior
The molecular structure of polymers, whether amorphous or semi-crystalline, plays a significant role on their behaviour during extrusion.
-
Amorphous Polymers:These materials, such as ABS, have a randomly organized molecular structure, which allows for lower shrinkage rates and makes them more predictable during extrusion and printing. Amorphous polymers soften gradually when heated to their glass transition temperature range, making them easier to control and more forgiving during the extrusion process. Their lack of crystalline regions makes these plastics transparent and also advantageous for applications requiring dimensional accuracy and visual clarity.
-
Semi-Crystalline Polymers: Semi-Crystalline Polymers: Polymers like PEEK and PA have a highly ordered molecular structure (crystalline regions) in some areas of their structure as well as amorphous regions. At the time of writing, there are no fully crystalline polymers commonly available. This combination of regions means these polymers have a glass transition temperature range on where the amorphous regions start to present mobility as well as a distinct melting point where its crystalline region goes from solid to completely melted. Depending on the percentage of crystallinity these polymers can present higher shrinkage rates upon cooling. In turn, these polymers often provide superior mechanical properties, such as enhanced stiffness and chemical resistance, but can be more challenging to extrude due to their rapid transition from solid to liquid. The precise control of cooling rates and temperature is crucial to avoid warping or dimensional inaccuracies in 3D printing and ovality and clogging in filament making.
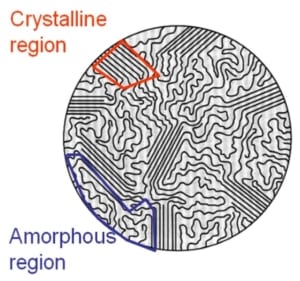
Thermal Conductivity and Cooling Rates
The thermal conductivity of polymers plays a pivotal role in their cooling rate during filament extrusion or 3D printing. Polymers generally have low thermal conductivity, meaning that heat dissipation during cooling is slow, potentially leading to thermal gradients within the printed part. This can cause differential shrinkage or internal stresses, which are especially problematic for large or complex 3D-printed structures.
For semi-crystalline materials, like POM (Acetal) or PA12, the cooling rate also directly affects the crystallization process. Controlling the cooling rate is critical to achieving the desired crystalline structure, which in turn affects the mechanical properties of the final part. Slow cooling promotes higher crystallinity, resulting in a stiffer and more dimensionally stable part, but can also increase the risk of warping if not carefully managed.
What is the Polymer Pyramid?
The Polymer Pyramid or as it is also referred to the Plastic Pyramid is a model used in 3D printing and filament extrusion that divides plastics into three main tiers based on their material properties. The three properties are Commodity Plastics, Engineering Plastics, and High-Performance Plastics. Multiple aspects are considered when grouping polymers like PLA, PEEK and others but mainly we look at the temperature tolerance, chemical resistance and mechanical strength of each plastic.
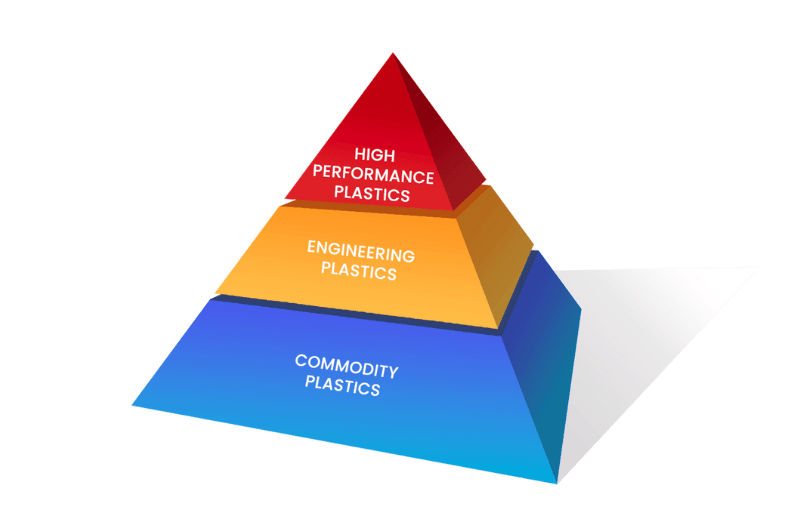
Tier 1: Commodity Plastics – Affordable and Accessible
Commodity Plastics form the foundation of the Polymer Pyramid, representing the most widely used and cost-effective materials in the industry. These polymers are ideal for high-volume applications due to their ease of processing, low cost, and availability. While they do not provide the high strength, chemical resistance, or thermal stability found in engineering or high-performance plastics, their value lies in their cost-efficiency and suitability for mass production.
Technically speaking, commodity plastics like Polyethylene (PE), Polypropylene (PP), Polystyrene (PS), and Polylactic Acid (PLA) exhibit lower melting points and moderate mechanical properties, making them easier to process at lower temperatures. This reduces energy consumption during filament extrusion and FDM (Fused Deposition Modeling) 3D printing, allowing for faster print speeds.
Thermal Stability and Cooling Considerations
Commodity plastics like Polyethylene (PE), particularly low-density polyethylene (LDPE), are known for their flexibility and impact resistance. While these properties make LDPE suitable for applications like bottle caps and plastic bags, controlling the cooling rates and thermal behavior during the printing process is crucial to avoid defects such as warping.
PLA is another popular material in this category, known for its ease of use and biodegradability.
Additives and Compounding for Enhanced Performance
While commodity plastics are cost-effective, their performance can be enhanced through additives or compounding. For example, colorants, plasticizers, and stabilizers can be added to improve properties such as appearance, flexibility, or UV resistance. These modifications make commodity plastics more versatile and suitable for a wider range of applications.
In some cases, blending polymers, a process that can yield co-polymers with properties that differ from the original plastics. For instance, PLA can be blended with additives to improve its heat resistance, making it suitable for more demanding environments.
For instance, PLA can be blended with additives to improve its heat resistance, making it suitable for more demanding environments.
Applications in 3D Printing
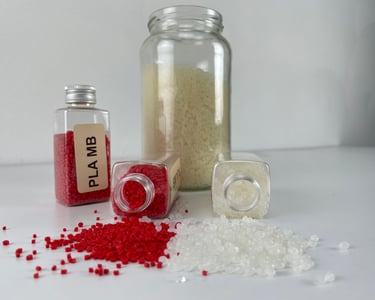
-
Polylactic Acid (PLA): A biodegradable plastic derived from renewable resources like corn starch. PLA is known for its ease of printing, low warping, and good surface finish, making it ideal for beginners to the 3D printing space. However, its low-temperature tolerance makes it unsuitable for high-heat applications.
-
High-Density Polyethylene (HDPE): Known for its strength-to-density ratio and chemical resistance, HDPE is used in food-safe applications, containers, and packaging. While it’s more challenging to print due to warping, HDPE is valued for lightweight, durable parts in automotive components and functional parts.
-
Polyethylene Terephthalate Glycol (PETG): PETG combines the strength of ABS with the ease of printing of PLA. It offers excellent chemical resistance, low shrinkage, and transparency, making it ideal for mechanical parts and prototypes. PETG is often used in functional components that require a balance between flexibility and durability.
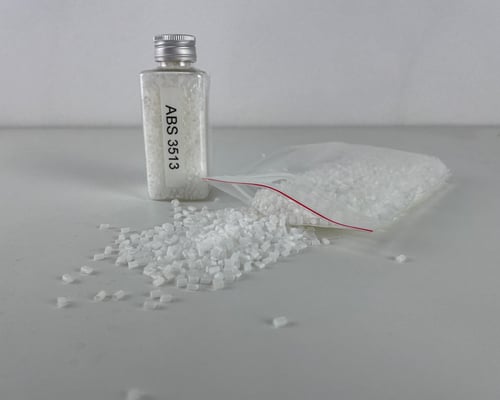
-
Acrylonitrile Butadiene Styrene (ABS): ABS is a strong, durable plastic used in automotive parts, toys, and consumer products. Known for its impact resistance and heat tolerance, ABS requires a heated bed during printing to prevent warping. It is ideal for functional parts and enclosures that need to withstand stress.
-
Polypropylene (PP): A flexible, chemically resistant plastic often used in 3D printing for hinges, containers, and functional prototypes. PP’s low density and resistance to moisture make it suitable for automotive and packaging applications, although it can warp without proper print settings.
-
Polystyrene (PS): High-impact polystyrene (HIPS) is commonly used as a support material in 3D printing due to its solubility in limonene. PS is also used in packaging, disposable products, and prototypes where cost-efficiency is important. However, it lacks the strength required for load-bearing applications.
-
Low-Density Polyethylene (LDPE): A flexible material with impact resistance, LDPE is used in applications like bottle caps and plastic bags. It’s not commonly used in 3D printing but can be suitable for specific applications requiring high flexibility.
Tier 2: Engineering Plastics – Performance and Durability
Engineering Plastics occupy the middle tier of the Polymer Pyramid, offering a balance between cost-efficiency and enhanced mechanical properties. With superior chemical resistance and thermal stability, engineering plastics are well-suited for structural components and industrial parts where reliability and durability are essential. These materials are designed for applications that require greater strength, durability, and thermal resistance than commodity plastics, making them ideal for high-performance environments.
Technically speaking, engineering plastics like Polyamide (PA), Polyoxymethylene (POM), Polycarbonate (PC), and Thermoplastic Elastomers (TPE) exhibit higher melting points and greater mechanical strength than commodity plastics, resulting in more consistent performance under stress. These polymers, including Thermoplastic Polyurethanes (TPU), also offer greater flexibility and rubbery behavior, making them suitable for flexible, impact-resistant parts. However, these materials often require higher processing temperatures and more precise control during extrusion and 3D printing.
.jpg?width=533&height=400&name=MicrosoftTeams-image%20(58).jpg)
Thermal Stability and Cooling Considerations
One of the advantages of engineering plastics is their ability to maintain dimensional stability under higher temperatures. For example, Polycarbonate (PC), widely used in automotive and electronics applications, offers high impact resistance and can withstand temperatures up to 120°C. However, elastomeric materials like TPE and PCL have lower melting points, with PCL being known for its biodegradability and low-temperature flexibility. PCL melts around 60°C, making it ideal for medical devices and biodegradable products, but its low melting point also means that parts may soften at elevated temperatures.
The cooling rate is critical for these materials. Rapid cooling can lead to internal stresses or crystallization issues that may affect the part’s mechanical properties. In the case of semi-crystalline polymers like PA or POM, controlling cooling temperatures ensures a uniform crystalline structure, contributing to long-term stability and reducing the risk of warping.
For TPU and TPE, cooling must be managed carefully to retain the material’s flexibility and rubbery texture without compromising surface quality. These materials often require specialized print settings and cooling profiles to avoid defects, ensuring consistent elasticity and smooth finishes in the final product.
Additives and Compounding for Enhanced Performance
Engineering plastics, including elastomers like TPU and TPE, can be further tailored for specific applications through additives or compounding techniques. Reinforcing materials like glass fibers or carbon fibers can be added to PA to improve its tensile strength and thermal resistance. These additives are particularly useful in applications where strength and rigidity are required, such as gears, automotive components, and machine parts.
Plasticizers are often added to elastomers like TPU to improve their flexibility and resilience, especially in applications like soft-touch handles or wearables. However, adding too much plasticizer can compromise the material’s mechanical properties, leading to issues like sagging or tearing during use.
For biodegradable polymers like PCL, the compounding process can involve the addition of biodegradable additives to enhance its performance in specific applications, particularly in the medical or eco-friendly packaging sectors. Stabilizers such as UV inhibitors can be incorporated into PC and TPU to protect them from degradation in outdoor environments, extending their useful life.
Applications in 3D Printing
-
Polyamide (PA): Also known as Nylon, PA is prized for its strength and flexibility. In FDM printing, it’s commonly used for functional prototypes, gears, and machine parts requiring high impact resistance. However, its tendency to absorb moisture from the air means it must be kept dry before and during the printing process to avoid defects.
-
Polycarbonate (PC): Known for its toughness and transparency, PC is used in FDM printing for protective covers, medical devices, and automotive components. Its high heat resistance makes it ideal for applications where durability under temperature fluctuations is essential, but PC requires higher nozzle temperatures and heated print beds to avoid warping.Additives such as glass fibers or carbon fibers can be added to PA to improve its tensile strength and thermal resistan
-
Polycaprolactone (PCL): With its low melting point (around 60°C), PCL is biodegradable and often used for medical applications like implants or biodegradable packaging.
-
Thermoplastic Polyurethane (TPU): Known for its flexibility and elasticity, TPU is often used in FDM for wearable devices, protective covers, and soft-touch components. TPU’s unique properties require fine-tuned print settings to avoid issues like stringing or oozing during printing, but when done correctly, it provides durable, flexible parts with superior wear resistance.
-
Thermoplastic Elastomer (TPE): Offering rubber-like flexibility, TPE is ideal for soft-touch applications, grips, and flexible parts like seals or gaskets. TPE can be challenging to print due to its high flexibility, but it is a preferred material for products requiring stretchability and impact resistance.
%20-%20instasize%20(1).png?width=500&height=400&name=MicrosoftTeams-image%20(17)%20-%20instasize%20(1).png)
Tier 3: High-Performance Polymers – Ultimate Strength and Stability
High-Performance Polymers sit at the apex of the Polymer Pyramid, representing the most advanced and durable materials available. These polymers are designed to withstand extreme conditions, offering unmatched mechanical strength, thermal stability, and chemical resistance. They are used in industries where performance under stress is non-negotiable, such as aerospace, automotive, medical devices, and industrial manufacturing.
Technically speaking, high-performance polymers like Polyetheretherketone (PEEK), Polyetherketoneketone (PEKK), and Polyetherimide (PEI) are known for their ability to maintain structural integrity in high-temperature and chemically aggressive environments. These materials offer superior mechanical properties, including high tensile strength, stiffness, and creep resistance, even at temperatures exceeding 250°C. However, their complex molecular structures, typically semi-crystalline, require precise control during extrusion and 3D printing to ensure optimal performance.
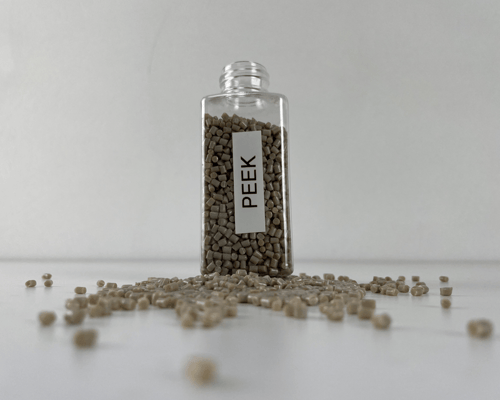
Thermal Stability and Crystallization
High-performance polymers like PEEK and PEKK are known for their exceptional thermal resistance, maintaining their mechanical properties even in environments exceeding 250°C. These polymers are often used in aerospace and automotive applications, where parts are exposed to extreme heat and require long-term durability. Their semi-crystalline nature contributes to their high melting points and excellent chemical resistance, making them ideal for use in harsh, chemically reactive environments.
The crystallization behavior of these materials plays a crucial role in their dimensional stability and mechanical performance. During the extrusion or printing process, careful control of the cooling rate is necessary to achieve the desired crystalline structure, which affects the final part’s strength and rigidity. Rapid cooling may result in amorphous regions, reducing the material’s mechanical properties, while slower cooling can promote crystallinity, enhancing the stiffness and heat resistance of the part.
In 3D printing, particularly with FDM technology, ensuring consistent cooling is essential to avoid warping or shrinkage, especially with high-performance materials like PEEK and PEI. PEI (Ultem), for instance, is widely used in medical devices and electrical components due to its flame retardancy and electrical insulation properties, but it requires high nozzle temperatures and careful bed adhesion techniques to avoid defects during printing.
Chemical Resistance and Long-Term Durability
High-performance polymers are also known for their chemical resistance, making them ideal for use in environments where parts may be exposed to corrosive chemicals or very acidic or basic environments. PEEK and PEKK, for example, are virtually impervious to hydrocarbons, acids, and alkalines, making them suitable for use in chemical processing equipment and medical implants. Their resistance to hydrolysis and radiation further extends their applications in biomedical and pharmaceutical industries.
The long-term durability of these polymers makes them ideal for parts that need to retain their properties over extended periods of time, even under mechanical stress and high temperatures. This includes applications in turbines, gears, and valves, where components are subjected to constant wear and chemical exposure.
Additives and Compounding for Enhanced Properties
While high-performance polymers already offer superior properties, they can be further enhanced through additives and compounding techniques. For example, carbon fibers or glass fibers can be added to PEEK to increase its tensile strength and stiffness, making it suitable for load-bearing applications. These reinforcements are commonly used in the production of aerospace components, automotive parts, and industrial machinery, where parts must withstand both mechanical stress and thermal cycling.
PEKK, a close relative of PEEK, offers similar mechanical strength and chemical resistance, but with greater processability and the ability to be fine-tuned for specific applications through compounding. PEI is often compounded with stabilizers to improve its UV resistance and thermal stability, making it a popular choice for outdoor applications and medical devices that require sterilization.
Applications in 3D Printing
%20-%20instasize.png?width=500&height=400&name=MicrosoftTeams-image%20(26)%20-%20instasize.png)
-
Polyetheretherketone (PEEK): Known for its exceptional thermal resistance and chemical stability, PEEK is used in aerospace, medical implants, and chemical processing equipment. Its high processing temperatures (around 360°C to 400°C) make it challenging to print, but its superior properties make it a top choice for high-performance applications where long-term durability is critical.
-
Polyetherketoneketone (PEKK): PEKK offers similar benefits to PEEK but with greater processability and the ability to fine-tune its crystallinity and mechanical properties. It’s often used in aerospace and automotive parts, where heat resistance and mechanical strength are paramount. PEKK is more flexible in cooling rates, making it easier to print compared to PEEK.
-
Polyetherimide (PEI): PEI, commonly known as Ultem, is widely used for electrical components, medical devices, and aerospace parts due to its flame retardancy, chemical resistance, and high dielectric strength. Its high glass transition temperature (around 215°C) makes it suitable for applications requiring thermal stability and long-term durability.
Conclusion
Selecting the right 3D printing material is crucial for achieving the best results in your projects, and the Polymer Pyramid simplifies this decision-making process. Whether you're using commodity plastics for rapid prototyping or high-performance polymers for industrial-grade applications, understanding key material properties like thermal stability, and mechanical strength will directly impact the quality and performance of your 3D prints.
At 3devo, materials specialists Are ready to support and advise you in every step of your filament development journey. We offer personalized consultations and comprehensive testing services to ensure your materials are optimized for filament production.
Beyond the Material Test (Melt Flow Index (MFI) Test), which helps you understand your polymer’s flow properties for just €50, we also provide a Filament Development Analysis. This service evaluates whether the produced filament is printable. Simply send us your material, and we'll deliver a detailed Filament Development Report on the quality and performance of the filament, helping you ensure it's ready for 3D printing.
Ready to take the next step? Book a free video meeting with one of our materials experts today. During this personalized consultation, we’ll assess your project's feasibility, discuss material compatibility, and introduce solutions like our Filament Makers and GP20 Plastic Shredder to support your innovation.
Let us help you transform your materials into high-quality filament for all your 3D printing needs. At 3devo, we know that every innovation requires a personalized approach, and we’re here to guide you through every stage of your project.
Start Your Project
If you're ready to explore your options, schedule a call with our team to get started.
Share this
- December 2024 (2)
- November 2024 (3)
- October 2024 (4)
- September 2024 (2)
- August 2024 (3)
- July 2024 (6)
- June 2024 (3)
- May 2024 (2)
- April 2024 (2)
- March 2024 (1)
- January 2024 (1)
- November 2023 (2)
- October 2023 (5)
- September 2023 (2)
- August 2023 (1)
- May 2023 (1)
- January 2023 (1)
- December 2022 (3)
- August 2022 (1)
- June 2022 (1)
- May 2022 (2)
- April 2022 (2)
- March 2022 (7)
- February 2022 (2)
- January 2022 (3)
- December 2021 (3)
- November 2021 (3)
- October 2021 (2)
- September 2021 (3)
- August 2021 (3)
- July 2021 (2)
- June 2021 (1)
- March 2021 (1)
- October 2020 (1)
- June 2020 (1)
- May 2020 (1)
- April 2020 (4)
- November 2019 (1)
- July 2019 (2)
- June 2019 (1)
- May 2019 (1)
- March 2019 (1)
- November 2018 (1)
- September 2018 (1)
- January 2018 (1)
- October 2017 (1)
- September 2017 (1)
- July 2017 (1)
- June 2017 (1)
- May 2017 (1)
- January 2017 (1)
- December 2016 (3)
- November 2016 (2)
- October 2016 (1)
- May 2016 (2)
- August 2015 (2)
- July 2015 (1)